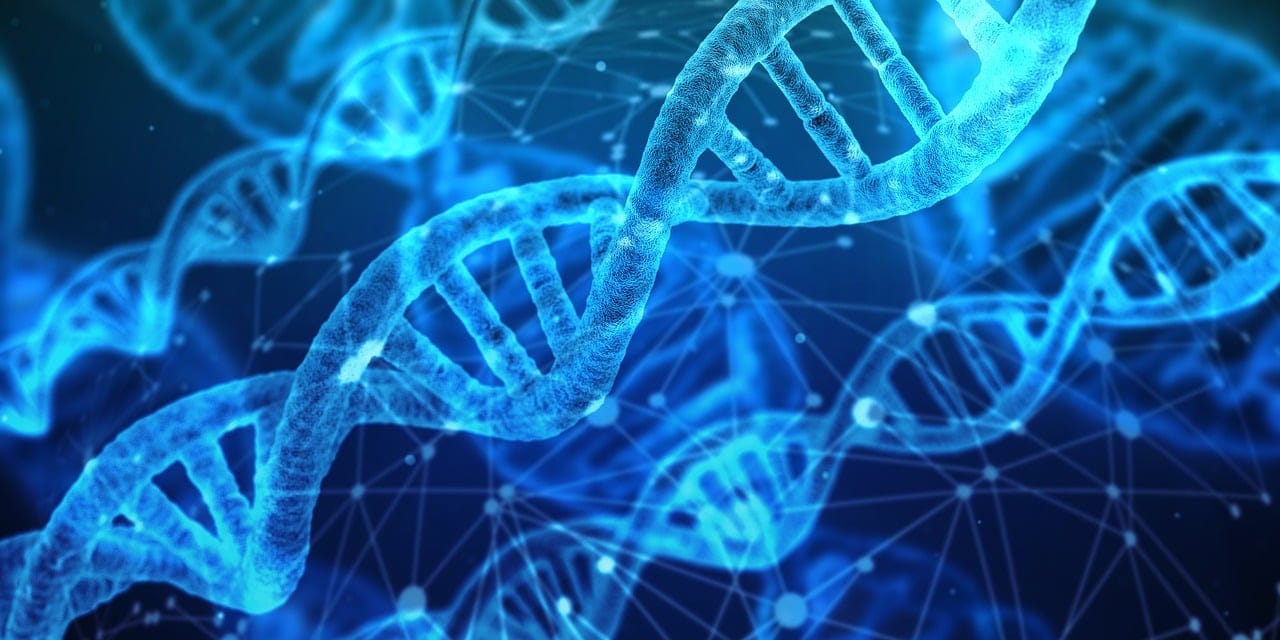
In vivo gene editing: The medicine of the future
The holy grail of genetic therapy has arrived.
The mountain of progress is steep and arduous, but we're still climbing it, one step at a time. Through the power of science, we're creating the miracle cures our ancestors implored the gods for.
A new paper could represent one giant step forward in this ascent. The holy grail of gene therapy is in vivo genetic editing: changing the DNA of cells inside our bodies. That's what a team of scientists has done.
The promise of base editing
You've likely heard of CRISPR, the revolutionary gene-editing technology derived from nature.
CRISPR is a proto-immune system discovered in bacteria. It encompasses a set of genetic sequences preserved from past viral infections, paired with DNA-cleaving enzymes that can be "loaded" with one of these sequences. Like a police officer checking suspects against a wanted poster, the CRISPR enzyme seeks out genetic material and compares it to its onboard guide sequence. If it finds a match, it cuts the DNA to pieces.
Bacteria evolved this system to destroy invading viruses, but we clever humans figured out another use for it. We can load the CRISPR enzyme with the sequence of a defective gene that causes a disease. If delivered into a cell, it targets the incorrect gene and cuts it out.
The cell has repair mechanisms to fix broken DNA strands, but they work best from a template. To take advantage of this, scientists can administer the CRISPR enzyme together with a corrected DNA sequence that matches the cut ends. The hope is that the cell will stitch in the new sequence where we want it to go.
This usually works, but the repair mechanisms aren't perfect. Sometimes, they create random DNA changes at the location of the break. That's undesirable, since these changes can lead to cancer or other problems down the line.
Scientists are continuing to improve and refine CRISPR technology. One of the newer techniques they've invented is called base editing. It changes one DNA letter at a time without cutting the DNA strand. The changes permitted by this technique are more limited, but they're safer and more precise.
In theory, base editing allows us to target and fix genetic diseases caused by a single DNA base change (known as a single-nucleotide polymorphism, or SNP). While that isn't every genetic disease, it includes some of the most common ones.
No user-serviceable parts
Whatever technique we use, the biggest problem is how to deliver it where it's needed. Changing DNA isn't as easy as editing a document.
Evolution hasn't designed our bodies to contain user-serviceable parts. Our DNA doesn't have a convenient central location where we can get at it. It exists in trillions of copies distributed throughout all the cells in our body. Within each cell, it's wrapped up within the nucleus, nested within the cell membrane, and guarded by the immune system. To rewrite our genetic program, we have to find a way to bypass the body's defenses and deliver the DNA-altering enzymes to hit billions of targets at once.
This is a staggeringly complex task. It's no coincidence that the first diseases cured by genetic therapy are ones where it's possible to alter cells outside the body in a controlled lab environment. For example, we've used CRISPR to cure sickle cell disease and related blood disorders. Scientists harvest stem cells from a person's bone marrow, perform the genetic edit, and transfuse the modified cells back into their body.
Obviously, this approach doesn't work for genetic diseases that affect organs and other solid tissues. To cure those, we have to alter cells inside the body. That's an even more difficult problem, and it seemed like an insurmountable hurdle.
Until now.
Saving KJ's life
The crisis that spurred this breakthrough was a baby born in 2024 with a rare and deadly genetic disorder called CPS1 deficiency.
When the liver breaks down protein, it creates ammonia as a waste product. Normally, the body disposes of this through a chain of reactions called the urea cycle, converting ammonia to nontoxic urea, which we excrete in urine.
In CPS1 deficiency, a mutation disables a key enzyme of the urea cycle. The body can't get rid of ammonia, which builds up to toxic levels in the blood (hyperammonemic crisis). It can be treated with a liver transplant, but babies born with this disorder often die before they're old enough for the surgery.
KJ, the baby with this disorder, was in this dire situation. He was put on liver dialysis, a low-protein diet and a nitrogen-binding blood medication, but all these were only stopgaps. His health continued to worsen, and he was added to the transplant list at five months old.
Time was short, and with KJ facing brain and organ damage, his parents and doctors decided to try an experimental treatment rather than waiting for a transplant. The doctors sequenced his genome to determine the exact mutation he had, then designed a personalized base-editing therapy. Rather than using a virus to carry the gene editor, which can provoke a harmful inflammatory response, they encapsulated it in lipid nanoparticles that are taken up by cells and release their contents into the interior.
They engineered human cell lines in a petri dish to carry the same mutation, and dosed them with the gene editor to prove that it altered the DNA as intended. They tested it on mice and monkeys for safety and efficacy. Then, in February 2025, when KJ was six months old, they infused the gene editor into his body—and held their breath.
Almost immediately, there were signs that the treatment was working. KJ's blood ammonia levels fell, and his weight started to increase. He caught a viral infection, which he recovered from without suffering a hyperammonemic crisis. Doctors were able to increase his dietary protein levels and decrease his dose of the nitrogen-binding drug without any adverse effects.
Three months later, KJ is alive, healthy and growing. It's too soon to tell if this is a lifelong cure—but all the indicators look good. The full scientific report on this treatment was published this month in the New England Journal of Medicine.
KJ was the first to benefit, but he won't be the last. There are more studies coming down the pipeline that use gene editing for treating disorders of the lungs, the heart, and more.
If this approach bears out, we'll be entering into a golden age of personalized genetic medicine. As this technique moves from revolutionary to routine, inherited diseases that were once killers will become curable. We can banish cancer, cystic fibrosis, Huntington's disease, Tay-Sachs disease, muscular dystrophy, hemophilia, and many more. We'll become masters of our own biology, no longer suffering the cruel whims of chance.